Research
Research at Jarillo-Herrero Group is focused on quantum transport in two-dimensional (2D) van der Waals materials and their heterostructures, aiming to uncover new quantum phenomena and develop novel electronic devices. In particular, as the birth place of magic-angle twisted bilayer graphene (MATBG), Jarillo-Herrero Group pioneers and is expertise in the field of “twistronics”.
General Reading of Review Articles:
-
-
- The electronic properties of graphene. Rev. Mod. Phys. 81, 109 (2009)
- Electronic transport in two-dimensional graphene. Rev. Mod. Phys. 83, 407 (2011)
- Van der Waals heterostructures. Nature 499, 419–425 (2013)
- Colloquium: Topological insulators. Rev. Mod. Phys. 82, 3045 (2010)
- Berry phase effects on electronic properties. Rev. Mod. Phys. 82, 1959 (2010)
- The marvels of moiré materials. Nature Reviews Materials 6.3 (2021): 201-206.
-
Next Generation Moiré Quantum Matter
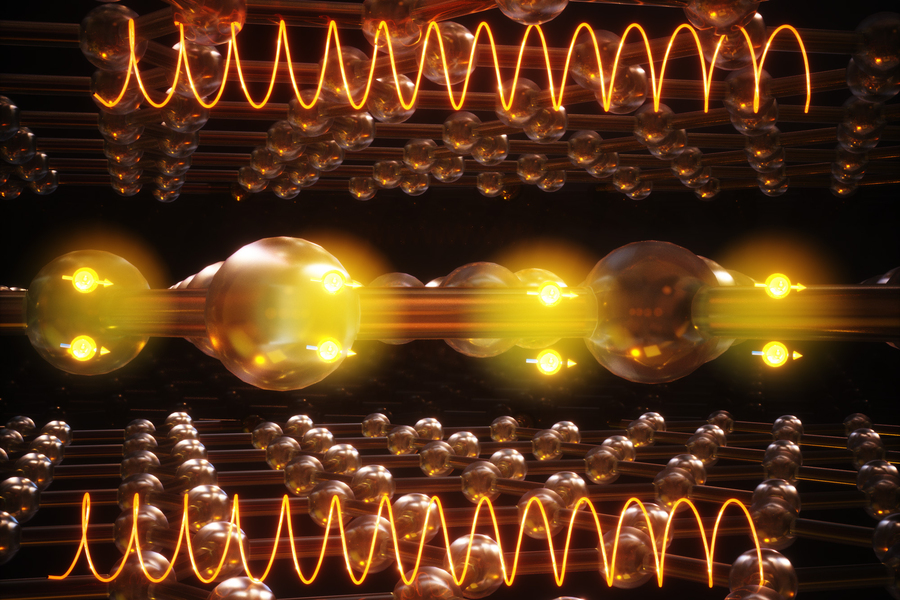
Our lab focuses on the fascinating world of moiré materials, where the twist angle or lattice mismatch between adjacent 2D atomic layers gives rise to moiré patterns. Since our groundbreaking discovery of correlated insulating states and superconductivity in magic-angle twisted bilayer graphene (MATBG) in 2018, the field of moiré materials has experienced explosive growth. This burgeoning area of research has unveiled a plethora of exotic phenomena in various moiré systems, including orbital magnetism and quantum anomalous Hall states, strange metal phases, generalized Wigner crystals, and fractional Chern insulators.
Two of the most exciting characteristics of moiré materials are their high tunability and the frequent coexistence of both strong correlations and non-trivial band topology. Motivated by the desire to uncover and understand novel correlated and topological phenomena, our lab is continuously dedicated to studying new generations of moiré structures. A promising direction is to explore systems beyond pairs of graphene layers. In recent years, we discovered magic-angle twisted trilayer graphene (MATTG), which exhibits strong-coupled superconductivity and re-entrant superconducting phases, suggesting an unconventional origin in spin pairing. Our subsequent work on 4- and 5-layer alternating twisted graphene demonstrated that magic-angle multilayer graphene forms a family for robust superconductivity.
More recently, our works on unequal-angle twisted trilayer graphene (TTG) and helical TTG pushed beyond a single moiré pattern for the first time. We discovered that unequal-angle TTG, with its quasiperiodic nature, hosts correlated states and superconductivity, while helical TTG relaxes into a polycrystalline structure, forming a mosaic of domains with varying Chern numbers. These findings offer new insights into designing next-generation moiré quantum materials for exploring correlated and topological physics.
Our recent articles about the topic:
- Unconventional superconductivity in magic-angle graphene superlattices. Nature 556, 43-50 (2018)
- Correlated insulator behaviour at half-filling in magic-angle graphene superlattices. Nature 556, 80-84 (2018)
- Tunable strongly coupled superconductivity in magic-angle twisted trilayer graphene. Nature 590, 249-255 (2021)
- Robust superconductivity in magic-angle multilayer graphene family. Nature Materials 21, 877–883 (2022)
- Superconductivity and strong interactions in a tunable moiré quasicrystal. Nature 620, 762–767 (2023)
- Topological bands and correlated states in helical trilayer graphene. Nature Physics 21, 239–244 (2025).
Ferroelectricity in 2D and its Applications
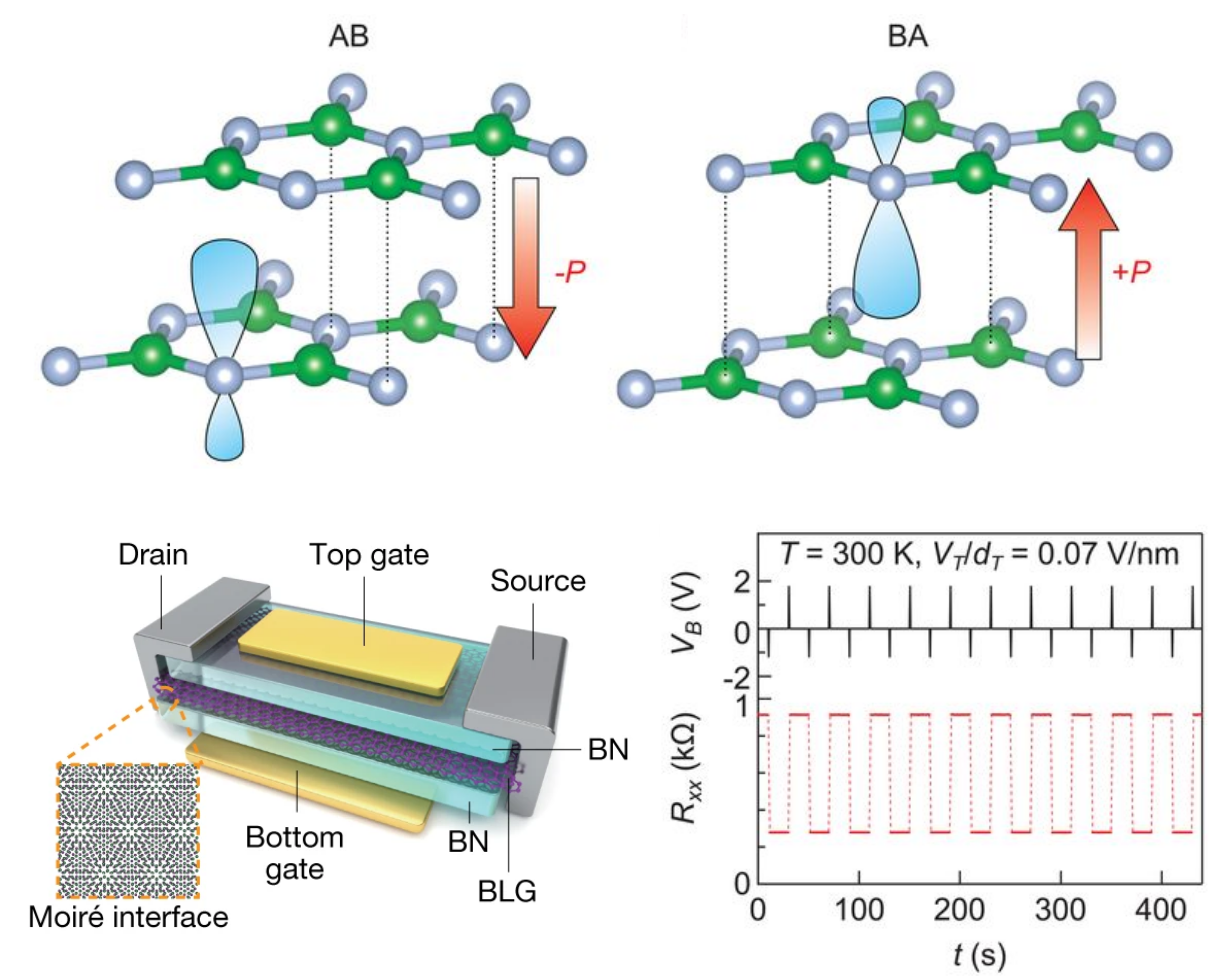
2D ferroelectric materials with atomic thickness and robust polarization provide an important building block for functional devices. Recently, our experiments revealed ferroelectricity in parallel-stacked or twisted hexagonal boron nitride (hBN) and transition metal dichalcogenides (TMDs). By building ferroelectric field-effect transistors, these material platforms demonstrated robust and configurable ferroelectric switching from cryogenic to room temperature, paving the way for a new generation of ultra-compact, endurable, and energy-efficient memory devices.
In another family of 2D materials, namely, asymmetric graphene/hBN moiré heterostructures, unconventional ferroelectricity emerges. These devices enable non-volatile injection of charge carriers that modulates the conductance. We have demonstrated that this type of ferroelectricity can be used to switch the correlated and superconducting states in MATBG in a bistable way, which could serve as a new type of cryogenic memory element.
Another exciting application of interfacial ferroelectricity is in the field of neuromorphic computing. The inherent tunability and non-volatility of asymmetric graphene/hBN moiré heterostructures make them ideal for mimicking the synaptic functions of biological brains. Our recent work reported room-temperature operation of a low-power (20 pW) moiré synaptic transistor, which realizes diverse biorealistic neuromorphic functionalities.
Our research unveiled the unique properties of 2D ferroelectric materials. Combined with their scalability and compatibility with existing semiconductor technologies, they hold great promise for revolutionizing memory storage, neuromorphic computing, and beyond.
Our recent articles about the topic:
- Unconventional ferroelectricity in moiré heterostructures. Nature, 588, 71–76 (2020)
- Stacking-engineered ferroelectricity in bilayer boron nitride. Science 372, 1458-1462 (2021)
- Interfacial ferroelectricity in rhombohedral-stacked bilayer transition metal dichalcogenides. Nature Nanotechnology 17, 367–371 (2022)
- Electrical switching of a moiré ferroelectric superconductor. Nature Nanotechnology 18, 331–335 (2023)
- Moiré synaptic transistor with room-temperature neuromorphic functionality. Nature 624, 551–556 (2023)
- Moiré band structure engineering using a twisted boron nitride substrate. Nature Communications 16, 178 (2025).
Transport Measurement at Ultra-Low Temperatures
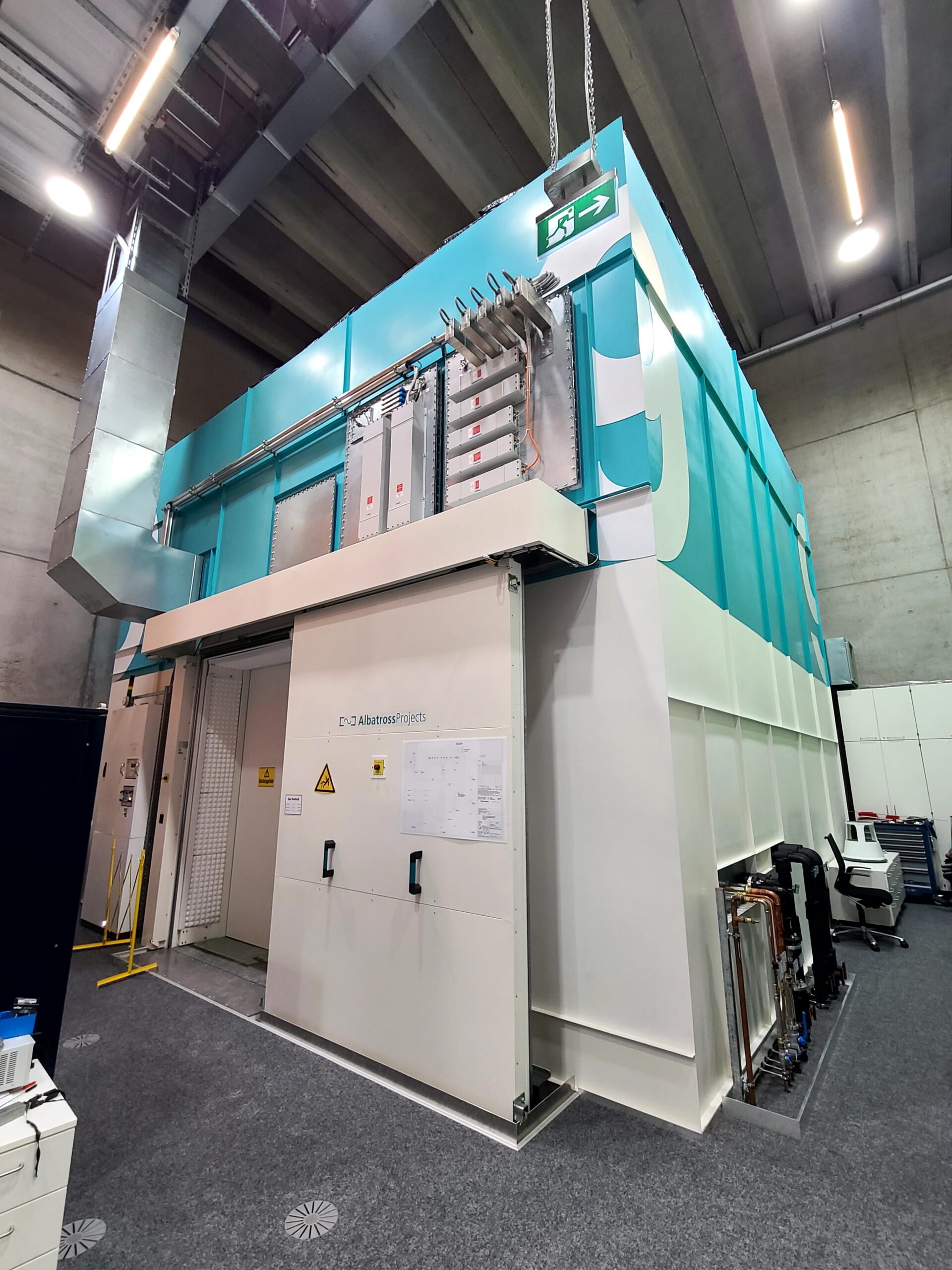
The observation of fragile quantum phenomena typically requires extremely low temperatures, where thermal activation is lower than the characteristic energy of focused states. Commercially available dilution refrigerators can reach temperatures as low as 10 millikelvin. However, at these ultra-low temperatures, the electron-phonon coupling between electrons and the substrate decreases, causing the electron temperature within the samples to be several times higher than the fridge’s nominal temperature.
To address this challenge, we have incorporated cryogenic low-pass filters into our dilution refrigerators to reduce radiofrequency noise. These filters include commercial RC and LC filters, as well as homemade distributed filters.
Recently, electron temperatures below 1 millikelvin have been reported in nanoelectronic devices utilizing adiabatic nuclear demagnetization. Inspired by these advancements, we are building a system to cool two-dimensional materials, such as multilayer graphene, down to sub-millikelvin temperatures. To pursue this goal, we have been setting up a dedicated lab at the Max Planck Institute for Solid State Research. This new facility will enable us to explore and understand novel quantum phenomena in these materials at unprecedented low temperatures.